PhD Opportunities
Possible research topics to be undertaken in the Biomedical Engineering Division of the James Watt School of Engineering are given below. If you are interested in any of these projects, you should email the prospective supervisor associated to the chosen topic for discussing your intentions (see contact details by following the topic link).
Alternatively, you are welcome to identify a different project topic within any of our research areas and email your project proposal to the Head of Division, Prof Huabing Yin (Huabing.Yin@glasgow.ac.uk), who will direct you towards a prospective supervisor with expertise in that area.
Single-Cell Raman Platform for Decoding Fatigue in Chronic Diseases - Dr. Jiabao Xu
Supervisor
Description
Fatigue is a common experience for every one, but when it persists severely for over six months, it's defined as chronic fatigue. This intense fatigue is a symptom seen in various chronic diseases like Parkinson’s disease, chronic heart failure, and rheumatoid arthritis. Often considered a challenging aspect of these conditions, fatigue is associated with functional impairment. The increasing prevalence of multi-morbidity, where individuals face multiple chronic conditions simultaneously, adds complexity to our understanding of the relationship between chronic diseases and fatigue.
In our lab, we aim to develop a single-cell platform aiming for understanding single-cell metabolism and disease. Single-cell Raman spectroscopy presents a label-free, minimally invasive, and multiplex analytical approach in the clinical realm. Analysing cells from patients through spectroscopy provides diagnostic and monitoring potential for future healthcare, allowing for swift disease diagnosis through specific spectral markers or signatures (Figure).
Potential PhD projects include:
- Development of a single-cell Raman-activated sorting platform for accurate targeting of immunometabolism in fatigue-related chronic diseases
- Decoding fatigue in psoriatic arthritis patients using Raman spectroscopy and metabolomics
- Artificial intelligence-aided spectroscopic diagnosis of myalgic encephalomyelitis/chronic fatigue syndrome (ME/CFS), long COVID, and chronic Lyme disease.
We are looking for PhD candidates with a passion in multidisciplinary subjects including advanced microscopy and spectroscopy, clinical science, machine learning and bioengineering. During the PhD, the student will be encouraged to collaborate with colleagues and clinicians in School of Infection and Immunity at UoG, University of Oxford and Peking University People’s Hospital.
Contact: Jiabao.Xu@glasgow.ac.uk
Biological inspired control and machine learning for clinical rehabilitation and engineering systems - Dr. H. Gollee
Supervisor
Description
Explaining how humans control movement and posture has been of interest to scientists and engineers for a long time, with controversy remaining about the nature of human motor control, and how this is related to our ability to adapt and to learn. One approach which we have developed in recent years, is based on a combination of open-loop predictive control and intermittent closed loop control, termed intermittent control. Questions which we currently investigate include
- mechanisms for human-like adaptation and learning,
- the role of data-driven methods such as AI and machine learning in an intermittent control structure, and
- the use of intermittent control to improve joint human-machine interaction, for example for users with special needs.
We are applying the insights gained from these developments in rehabilitation approaches, primarily based on neuro-prostheses (using electrical muscle stimulation). Since rehabilitation is a process of relearning, understanding how humans learn provides a basis for designing novel rehabilitation approaches. We are part of the Scottish Centre for Innovation in Spinal Cord Injury (http://www.scisci.org.uk) and have a long standing clinical research link with the Queen Elizabeth National Spinal Injuries Unit in Glasgow which enables us to evaluate these concepts in clinical experiments. Applications include
- abdominal electrical stimulation to support respiratory function,
- optimisation of stimulation parameters using novel patterns, and
- ultrasound imaging of muscle for functional diagnosis and optimisation of interventions.
The successful applicant should have a background in biomedical engineering and / or control engineering and machine learning, and an interest in interdisciplinary research involving control engineering and human physiology.
Contact: Henrik.Gollee@glasgow.ac.uk
Ultrasonic wave sensing of early-stage material degradation - Dr. A. Demčenko
Supervisor
Description
Within this programme, PhD candidates will have the opportunity to align their research with their personal interests by selecting a specific application or industry sector for ultrasonic wave sensing of early-stage material degradation.
Contact: Andriejus.Demcenko@glasgow.ac.uk
Bioinspired haptic electronics for neuro-prosthetics - Dr. H. Chen
Perceiving and responding to our surroundings is a fundamental function for us. Yet, illness, accidents, or aging can impair sensorimotor function, profoundly affecting daily activities. Simultaneously, there is a drive for scientific breakthroughs enhancing natural motion sensing abilities. Thanks to advances in material science and electronics, integrating cutting-edge device capabilities into the human body to solve above issues becomes possible.
Our group focuses on human tactile technology and study it from three angles:
1) Development of Innovative Functional Materials to ensure biocompatibility while harnessing biomimetic characteristics.
2) Design of Advanced Wearable Devices to replicate the body's innate sensing mechanisms and restore the body's sensor-motor functions.
3) Integration of Feedback Systems and Behavioral Analysis to promote the development of our discoveries towards clinical applications.
We are looking for highly motivated PhD candidates with a passion for multidisciplinary subjects including materials science, sensor & actuator design, advanced manufacturing and neuroengineering. Potential PhD projects include:
- Bioinspired physical-physiological sensors to mimic the perception function of human hands
- Multi-modes (mechanical, electrical and biochemical) tactile stimulation to artificially generate the sensor of touch
- Multi tactile perception (softness, roughness, friction and temperature) fusion
Contact: Haotian.chen@glasgow.ac.uk
Nanophotonic Devices - Prof. A. Clark
Nanophotonic Devices Group
Our research seeks to develop cutting-edge nanotechnologies for practical applications.
Potential PhD projects include:
- Artificial tongue sensors for industrial, diagnostic, and environmental applications.
- Photonic metasurfaces for lensing, holography, and communications.
- Transformable colour nano-surfaces for security and imaging.
- DNA origami as a self-assembly tool for applications in sensing, therapeutics, and materials engineering
- Engineered Molecular Surfaces to gain insight into the mechanisms of RNA Splicing
You would be joining a vibrant multi-disciplinary research team and would have the opportunity to work closely with academic and industrial collaborators across the world.
This position is an excellent opportunity for a student with a background in either engineering, physics, chemistry or biology to work at the forefront of nanotech research.
More information at:
3D bioprinted scaffold : therapeutic approach for muscle loss - Dr. O. Dobra
Supervisor
Description
We are interested in developing novel piezoelectric constructs embedded in 3D hydrogel systems to reconstruct tissues such as muscle and others. This new class of functional materials, based on piezoelectric biocompatible and biodegradable polymers, will be able to stimulate cells mechanically and electrically. Combined with a protein-based hydrogel that efficiently presents growth factors, we aim to develop a dynamic functional environment similar to the native muscle tissue. We expect to promote faster growth of the muscle and restore tissue functionality that surpasses the current strategies that make use of passive materials. This material has a high potential to be translated into clinics as well as being used beyond the clinical industrial activity.
The project will be developed within the Centre for the Cellular Microenvironment, a multidisciplinary research centre, with access to cutting-edge technologies and equipment such as Brillouin microscopy and 3D bioprinting.
Contact: Oana.Dobre@glasgow.ac.uk
Wearable Biomedical Sensors for Personalised Healthcare - Dr. M. Amjadi
Supervisors
Description
In the current medical practice, the assessment and diagnosis of diseases relies on medical history checks, clinical examinations, and imaging techniques, which are expensive and restricted to geographical access to healthcare centres. In recent years, soft and wearable biomedical sensors have been extensively explored due to their potential for continuous, remote, and personalised monitoring of vital signs, such as respiratory rate, pulse rate, body temperature, and blood pressure. Due to their skin-like and conformal nature, these devices can provide long-term healthcare monitoring without disrupting the patients’ routine daily activities. Despite significant progress in this field, the full deployment of wearable sensors in practice remains a grand challenge given their manufacturing complexities, data collection and signal processing roadblocks, ethical considerations, and poor durability under large mechanical deformations.
Our group undertakes multidisciplinary research to address the aforementioned challenges. We develop novel functional nanocomposite materials and structures by incorporating micro/nanoscale materials (e.g., carbon nanotubes, nanowires, and graphene) into stretchable elastomers and textiles to manufacture high-performance and durable sensors. Furthermore, we focus on digital manufacturing technologies including 3D printing and laser micromachining to propose low-cost and scalable manufacturing processes. Our group is also interested in exploring wireless systems and machine learning for data acquisition, communication, and signal processing.
We are always looking for talented and highly motivated PhD candidates interested in bioelectronics, wearable technologies, smart materials, soft robotics, and digital manufacturing. This is an exciting opportunity to work on a dynamic and interdisciplinary research topic that intertwines engineering, materials science, and healthcare technology. Examples of PhD projects include:
- Soft nanocomposite sensors for healthcare monitoring
- Soft and wearable sensors for human motion detection, sport performance monitoring, and rehabilitation
- Wearable multiplexed electronic skins capable of selective and simultaneous assessment of multiple physical, chemical, and/or biological signs
- Soft physical sensors (e.g., touch and temperature) for prosthetic devices
- Soft and wearable battery-free bioelectronics
- Machine learning in materials science
Contact: Morteza.Amjadi@glasgow.ac.uk
Integrated biosensing platform for early disease detection and companion diagnostics - Dr. C. Hu
Supervisor
Description
Biosensing platforms for early diagnostics have become more and more popular in recent years due to their low cost, portability, and ease of use. We are interested in developing an integrated biosensing platform with combined functions of blood filtering, plasma desalting, blood defibrination, and biosensing, with a range ofsensors, including ion sensitive field effect transistor, and avalanche photodiodes.
Our current projects include:
- Integrated microfluidic device for front-end sample preparation.
- Highly sensitive sensing platform for early cancer diagnosis.
- Low-cost portable companion diagnostic device for bleeding disorders.
- Multi-layer microfluidic device for drug screening tests.
- Microfluidic platforms with 3D structure for nerve injury and regeneration study.
Applicants are welcome to propose their own project in this research area as well.
The work is highly cross-disciplinary, with components in the areas of electronics, engineering, and biosciences, though candidates with other background may also apply to discuss topic which would suit best their skills and aspirations.
During the PhD, the student will learn advanced manufacturing methods, biosensing and other analytical sciences. The student will be encouraged to collaborate with colleagues in The Beaston Cancer Research Centre, and Glasgow Royal Infirmary.
Contact: chunxia.hu@glasgow.ac.uk
Robust machine learning for autonomous agents - Dr. B. Porr
Supervisor
Description

Contact: Bernd.Porr@glasgow.ac.uk
Advanced Single-cell technologies for diagnosis and cancer research - Professor H Yin
Supervisor
Description
A cell contains multi-layered information, ranging from genetics to chemical composition, physical properties and most importantly, biological functions. This individual heterogeneity has become a major challenge in clinical diagnosis and studying diseases. To address this problem, we have been developing advanced single-cell technologies for both cell sorting and analysis. Together with collaborators, we have exploited these tools in cancer research, bacterial resistance diagnosis, and drug screening.
Below are potential projects that we’d like to further develop around this research theme. Specifics can be designed upon discussion.
- Nanoscale thermal and mechanical biology. Temperature is fundamental to many cellular functions. However, direct thermal measurements of cells with nanoscale resolution have just been made possible through our recent development of aqueous Scanning Thermal Microscopy. This project will employ this innovative technology to measure local temperature and mechanical properties of cells, and so address unsolved questions in nanoparticle-mediated thermal therapy for the treatment of cancer.
- High throughput Raman activated cell sorting. Raman-activated cell sorting (RACS) has emerged as a powerful tool for ‘deep mining’ a biological system, revealing its heterogeneity as well as establishing links between cellular function and genetic makeup. Building upon our pioneering continuous RACS platforms in flow, this project will develop a high throughput system integrated with deep learning for early diagnosis of cancer and screening of therapeutics.
- Single-cell Microfluidics for rapid diagnosis. This project aims to establish a user friendly, sample-to-result microfluidic device for rapid pathogen diagnosis and antibiotic susceptibility tests in clinical setting. It involves working closely with our collaborators in hospitals and advanced manufacture.
These projects are cross-disciplinary and we welcome applications from motivated engineers, physicists, biologists and material scientists. The student will learn advanced manufacturing methods, imaging analysis and other analytical sciences. There will be many opportunities for s/he to travel and work alongside our collaborators.
Contact: Huabing.Yin@glasgow.ac.uk
Brain Computer Interface for Neurorehabilitation and Neuromodulation - Dr. A. Vuckovic
Supervisors
Description
Brain Computer Interface (BCI) technology provides information about brain activity in real time and translates that activity into actions of a computerised system. BCI can be used for communication, to control computer application or environment through brain activity. However BCI can also be used to train brain to work in a certain way (neuromodulation) as a part of a therapy or improvement of cognitive and motor functions in heathy people. BCI based neurorehabilitation is used for restoration of lost motor and sensory functions, caused by spinal cord injury and stroke.
The BCI group offers PhD projects ranging from applications for healthy, fit people to applications designed for patients. Research laboratories are available both at the University and at the Queen Elizabeth University Hospital, facilitating clinical applications.
Applicants can chose from a list of projects below or can propose their own project in the area of BCI.
1. Hybrid multiuser BCI
2. BCI and VR games
3. Creating synthetic EEG data for improving BCI performance
4. BCI with functional electrical stimulation for restoration of motor function
5. Patient self-managed BCI therapies
6. Neurofeedback therapies for neuropathic pain
7. Neurofeedback therapies for improved concentration and memory
8. “Neurodoping” of healthy people for improvement of motor or cognitive functions
9. BCI games for single and multiple users (healthy people or patients)
10. Machine learning strategies for BCI and quantitative EEG analysis
11. Quantitative EEG for early detection of neuropathic pain
12. BCI and neuromarketing.
Engineering or computing background is desirable though candidates with other background may also apply to discuss topic which would suit best their skills and aspirations.
Contact: Aleksandra.vuckovic@glasgow.ac.uk
Multiscale mechanobiology of breast cancer - Professor. D. Gourdon
Supervisors
Description
Background: The extracellular matrix (ECM) is a fibrillar scaffold that plays an important role in many physiological processes such as gene expression and in pathologies such as cancer. More specifically, cells interact with the ECM to regulate their migration, proliferation, differentiation, and even death. Fibronectin and collagen are two key proteins of the ECM that have been implicated in breast cancer progression. In fact, our group previously demonstrated that cancer-associated cells generate high quantities of both fibronectin and collagen with altered structural and mechanical properties that favour breast tumour growth.
Project: In this project, the student will learn freeze-casting techniques to generate fibronectin-collagen three-dimensional (3D) scaffolds with tuneable microarchitecture and mechanics that mimic either the healthy or the cancerous microenvironment. He/she will also learn Fӧrster resonance energy transfer (FRET) spectroscopy and atomic force microscopy (AFM) to monitor single protein structure and scaffold stiffness, respectively. These 3D platforms will then be utilized to investigate the effect of ECM structure and mechanics on cancer cells invasion and tumour growth. By tuning collagen and fibronectin properties, we should be able to regulate breast cancer cell functions including cell adhesion and proliferation, and thus, potentially prevent tumour growth and metastasis. Additionally, these 3D ECM-mimicking platforms will allow us to study cells over large volumes, i.e., for long-term cell culture, and therefore have potential applications in tissue engineering as well as regenerative medicine.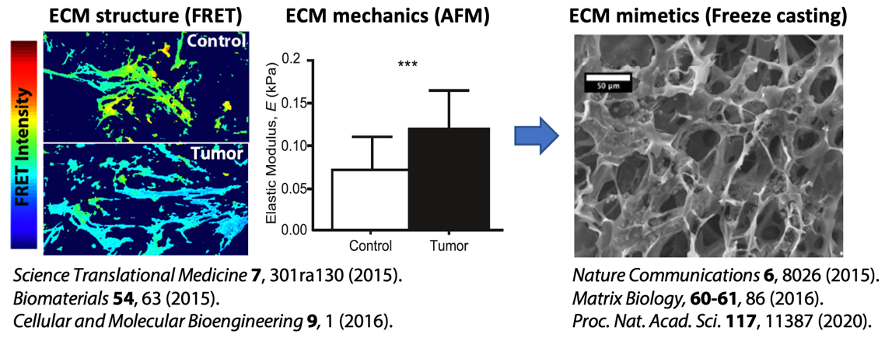
Candidate: This project is highly interdisciplinary, integrating approaches from engineering, biophysics, and life sciences, which will suit candidates with interests in biomaterials, microscopy/spectroscopy and mechanics.
Collaborations: During his/her PhD, the student will be encouraged to collaborate with colleagues in the Beatson Cancer Institute, the Physics Department of University of Glasgow, as well as with international collaborators providing opportunities to travel.
Contact: Delphine.Gourdon@glasgow.ac.uk; more info: www.gourdonlab.org
How do cells feel and channel mechanical stimuli? Methods and applications in cell mechanosensitivity - Dr. M. Vassalli
Supervisors
Description
Living cells continuously adapt to their environment, interpreting chemical and physical stimuli and transducing them into biological messages. Besides its key role in several physiological processes, this mechanism is still poorly understood. Understanding mechanotransduction will not only improve the knowledge of cell physiology, but also provide the basis for further investigating related disorders. Among all molecular actors of mechanotransduction, we are especially interested in the biophysics of the mechanosensitive ion channels Piezo1 and Piezo2. These molecules, recently identified as bona fide mechanosensors in mammalian cells, open up in response to locally applied force, providing a direct mechanism for converting mechanical stimuli in a downstream biochemical cascade.
Specific projects are available, either on the technological aspects of measuring single cell mechanosensitivity, or on the role of mechanosensing for cell physiology and pathology.
Figure: time lapse of a single cell responding to mechanical stimuli through the activation of mechanosensitive channels and the corresponding rise in calcium concentration, here encoded in colors
Contact: Massimo.Vassalli@glasgow.ac.uk
Microrheology of 3D cell culture gels - Dr. M. Tassieri
Supervisors
Description
It has been established that the mechanical properties of cell culture gels control the fate of cells. However, despite its importance, a one-to-one correspondence between gels' stiffness and cell behaviour is still missing from literature.
Preliminary rheological studies on hydrogels have revealed (i) that conventional bulk rheology may overestimate the stiffness of gels by up to an order of magnitude; and (ii) that the actual stiffness of gels could be determined by means of microrheology measurements, which are inherently performed at cells length scales.
The aims of this PhD study are
(i) to develop a 'gold standard' microrheology method for determining the viscoelastic properties of a variety of commonly used 3D cell cultures gels; and
(ii) to identify a one-to-one correlation between gels’ viscoelastic properties and cells’ fate.
Such yearned information would be pivotal, for instance, to the fight against cancer diseases; hence, the potential impact of this PhD study.
Contact: Manlio.Tassieri@glasgow.ac.uk
Point-of-care, low-cost medical diagnostic technologies for low-resource settings - Dr. J. Reboud
Supervisor
Dr. Julien Reboud; Professor Jonathan Cooper;
Description
Background: Creating Lab-on-a-Chip and biosensing devices for low-resource settings is an exciting new area of science. Applications encompass both diagnostics at home or the GP clinic in the UK, as well as in poor rural populations globally. The subject involves the use of advanced manufacturing technologies to produce accurate, sensitive and robust devices to detect and diagnose infectious diseases. The devices have a series of additional demands, requiring that they are easy to use, readily disposable and have minimal or no power requirements.
We have two particular interests:
- in creating ‘sentinel devices’ that are able to monitor multiple diseases in a specific area in a multiplexed format
- in informing personalised treatment by analysing drug resistance, which is becoming a crucial burden on the global scale.
Projects: We have developed two main technologies to overcome current limitations in ease of use and cost. One is linked to acoustics, via the use of surface acoustic waves to drive microfluidic manipulation of reagents on a disposable surface. With the help of advanced metamaterials and a mobile phone platform, we aim to integrate all complex manipulations involved in DNA-based assays onto a single device. We have already demonstrated this capability in detecting malaria, for which the analytical challenge lies in having the capability of measuring a single parasite-infected cell in a drop of blood, which might itself contain more than one million non-infected blood cells (by analogy, looking for a needle in a haystack). We work closely with parasitologists and epidemiologists who have field experience, working in rural Africa.
The second technology is based on paper. Paper is a cheap platform to develop advanced detection tools, which can be disposed by burning after use, making it ideal for low and high-resource scenarios. We have developed a platform based on the folding of paper matrices, akin to ‘origami’, to integrate molecular biology processing steps (DNA extraction, amplification, detection) into a single low-cost device. This platform was validated on clinical samples for the detection of malaria in Uganda, including speciation, an important factor for drug resistance.
All of these projects are also underpinned by essential quality control throughout the diagnostic process, from device manufacturing to result analysis. We have recently developed machine learning tools, powered through blockchain protocols to integrate the diagnosis into complex online clinical decision tools.
Candidate: The work is cross-disciplinary and we are equally interested in motivated microbiologists, engineers, parasitologists or physical scientists. The student, regardless of background, will learn advanced manufacturing methods and will train in analytical sciences. There will be many opportunities to interact with our collaborators working in parasitology.
Contact: Julien.Reboud@glasgow.ac.uk
Development of new bioactive hydrogels for therapeutic protein delivery during tissue regeneration - Dr. C. Gonzalez-Garcia
Supervisors
Description
Controlled delivery of biological molecules, such as growth factors, is a highly promising strategy to treat pathological conditions, including bone defects and skin lesions, as well as inflammatory, metabolic and neurological disorders. Carriers are often used to increase the retention of these molecules at the treatment site for enough time to allow cells to migrate to the area of injury and to stimulate cellular growth and differentiation, and drive regeneration. A major roadblock to effective protein therapeutics is the lack of biocompatible and injectable carriers that deliver growth factors locally with high bioactivity and suitable release rates.
We are interested in the design and development of new hydrogels, based on a natural polysaccharide, that act as bioactive carriers for cells and growth factors, promoting tissue regeneration processes. We will tune these hydrogels to mimic the physiological cues that cells find in vivo within native tissues in order to modulate mesenchymal stem cell differentiation towards different phenotypes, making the system suitable for different tissue engineering applications. These hydrogels will be produced in different configurations, including pre-formed microgels, prepared by microfluidics techniques, that can be envisioned to be simply injected into the body through minimally invasive surgery.
This system will be initially used for bone regeneration. However, the system will may be optimized for its translation to other applications such as wound healing and tissue repair. This is a multidisciplinary project that can be tailored to the candidate’s interests and will be developed within the Centre for the Cellular Microenvironment, with access to state-of-the-art technologies, including microfluidics and 3D printing.
Contact: Cristina.GonzalezGarcia@glasgow.ac.uk
Dissipative Materials for Regenerative Medicine - Dr. M. Cantini
Supervisors
Dr Marco Cantini;
Description
The PhD candidate will work on the design and development of bioinspired dynamic materials that mimic the spatiotemporal cues that cells encounter in vivo within native tissues. Cell-instructive materials for regenerative medicine are usually designed by simulating different aspects of healthy tissues, like their chemical and mechanical properties. However, most of the materials developed up to now to support cells in the repair of damaged tissues ignore the changing, viscous nature of the cells’ surroundings. Cells in vivo are in fact immersed in a dynamic, viscoelastic environment. They actively interact with it and remodel it, and these interactions help them to form and maintain specific tissues in our body.
We are interested in developing advanced smart materials that mimic the dynamic aspect of cells’ interactions with their native environment and exploit it to control cell behaviour (Cantini et al. 2019 Adv. Healthc. Mater.). Materials include viscoelastic hydrogels and dynamic biointerfaces, designed to guide stem cell differentiation to regenerate specific tissues, such as cartilage or bone. These synthetic microenvironments can also be used to control the behaviour of other cell types, e.g. cancer cells or neural cells, and as 2D or 3D models of healthy or pathological tissues.
The project can be tailored to the candidate’s interests and will be developed within the Centre for the Cellular Microenvironment, a multidisciplinary research centre where engineers, material scientists, cell biologists and clinicians work together, with access to state-of-the-art facilities, including atomic force microscopy and 3D printing.
Contact: Marco.Cantini@glasgow.ac.uk
Advanced dynamic materials for stem cell engineering - Prof. M. Salmeron-Sanchez
Supervisors
Professor Manuel Salemeron-Sanchez
Description
We are interested in engineering materials that recapitulate the properties of the Extracellular Matrix (ECM) to control stem cell phenotypes. Specific projects will be around designing novel systems that combine synthetic materials with proteins and growth factors that are responsive to external stimuli, such as light. Making materials responsive and switchable would add significant advantages over current static cell engineering strategies as would allow better, more precise - temporal control, of material properties (such as mechanical properties) to modulate stem cell responses. Another line of research, within this theme is the combination of synthetic biology and genetic engineering with biomaterials. We are interested in designing living materials, based on genetically engineered non-pathogenic bacteria that can respond to external triggers and provide stem cells with different stimuli at different time points.
Specific projects can be designed in dependence of the interests of the PhD candidate. For example, similar strategies can be used to control other types of cells (e.g. cancer or immune cells). All projects can incorporate state-of-the art technologies to engineer 3D microenvironments such as microfluidics and 3D bioprinting.
Keywords: biomaterials, extracellular matrix, growth factors, cell engineering, synthetic biology, genetic engineering.
Modelling and Simulation in Drug Discovery and Drug Delivery - Dr. S. McGinty
Supervisor
Description
In recent years, there has been move away from the testing of drugs in static 2D in vitro cell culture systems. More realistic systems that include dynamic flow and 3D structures of cells are increasingly being utilised due to the need to devise in vitroassays that are more predictive of in vivo behaviour. Such systems have the potential to significantly speed up drug development and lower the exorbitant costs involved with bringing a new drug to the market.
We are interested in developing modelling and simulation tools to help understand how cells respond to the varying mechanical, biological and chemical cues introduced in these more complex systems and to guide experimental protocols for drug testing in a variety of application areas including liver toxicity testing and parasitology.
We are also interested in better understanding the performance of devices which deliver drugs. Examples include drug-eluting stents for the treatment of coronary heart disease, therapeutic contact lenses for relieving eye problems and transdermal patches for delivering medication to the skin. We are interested in developing modelling and simulation tools to help understand why some devices fail; to more rationally design new drug delivery devices; to provide guidance to clinicians and; to assist with the regulatory approval process.
Contact: Sean.Mcginty@glasgow.ac.uk
Developing organs-on-a-chip using nanofabrication and AI - Prof. N Gadegaard
Supervisor
Description
The research group is combining nanofabrication technologies with computer based models to design next-generation biomaterials. The computational power available today has enabled us to make use of more complex computing science tools such as machine and deep learning. These tools can be used in an automated fashion to extract detailed information from microscopy images but can also be used to design better and smarter biomaterials. Using data from image analysis and molecular biology, the datasets can then be used to establish computational models for biomaterial design and physiological function for the desired target tissue/organ.
The research group extensively use the James Watt nanofabrication Centre to manufacture the nanoscale materials for the biological experiments. There is a rapidly growing demand to develop more physiological tools and models for drug testing. The majority of the tools available today are not realistically recapitulating the in vivophysiology of the human body. With the knowledge gained from our computer models and biological experiments, we develop the microphysiological environments to support different organs or tissues, e.g. immune system, liver, pancreas, fat or muscle. These are key organs in drug testing as well as understanding a number of vascular, metabolic or diabetic conditions. We develop the organs with local and international collaborators using using human induced pluripotent stem cells (hiPSC).
Projects exemplars (but not exclusive):
- High-content image analysis using machine and deep learning algorithms
- Developing AI and deep learning computer models for nanoscale biomaterials design
- Using information from computer models to design optimal environments for organs-on-a-chip
Contact: Nikolaj.Gadegaard@glasgow.ac.uk
New advanced medical imaging technologies - Pr. J. Cooper
Supervisor
Description
Background: Imaging and microscopies underpin both clinical diagnosis and basic biomedical research, studying disease processes, tissue regeneration and development.
Challenges: Our group is interested in developing new microscopy techniques to both increase the amount of information generated, but also reduce the cost and need for technical expertise. One current challenge involves developing new forms of microscopy that are able to determine the spatial location of biomarkers of diseases, such as arthritis, deep within the body to analyse the resulting structural changes within tissue and bones, which also include new therapeutic methods for restoration of organs and bone (e.g. stem cell differentiation).
A second challenge is to be able to perform advanced microscopy on low-cost, low-power platforms. Together with our collaborator Professor Ozcan (UCLA, USA), we have developed a new method that uses acoustic forcing of liquid samples to create optical components such as lenses directly within the sample. This allows us to integrate complex lens-free holography microscopy onto a mobile phone platform.
Projects: We aim to develop new imaging tools to
- probe deep into hard and soft tissues using highly innovative application of Raman spectroscopy. This will allow us, for the first time, to study non-invasively and quantitatively, the dynamics of biological processes at a molecular level, in real time. Such a tool will be used in the study of disease as well as during the regeneration of bone during therapy for example.
- Harness the interactions between sound and light, where acoustic fields are able to shape light wavefronts and allow a step-change in performance, from super-resolution to large field of view for example. These concepts will in turn enable to detect micro and nano-particles (such as viruses and bacteria) in biological fluids, thus enabling rapid diagnostics at the point-of-care (and in the field) on mobile phones.
Candidate: These projects are is highly interdisciplinary, involving the integration of approaches and methods traditionally found in Engineering, Physical Sciences and the Life Sciences. Consequently, it may, for example, suit candidates with an interest in microscopy and optics, instrumentation photonics.
Collaborations: During the PhD, the student will be encouraged to collaborate with colleagues in The Beaston Cancer Research Centre. We are also part of an international network of researchers including partners in NUS Singapore, Beijing Institute of Technology, Johns Hopkins USA, UCLA, Toronto and Harvard, providing the successful candidate with the opportunity to travel.
Contact: Jon.Cooper@glasgow.ac.uk
Cellular mechanics of cell differentiation and tissue growth (Mechano-tissue Engineering) - Prof. T Franke
Supervisors
Description
Mechano-biology has emerged as an important field in cell and tissue mechanics. It is a key factor in a wide range of biological processes including cell mobility, cell division and cancer. In tissue engineering, the key role of mechanics of the extracellular matrix (ECM) for cell fate has been recognized and viscoelastic properties of the ECM trigger stem cell differentiation into various linages. Naïve MSCs, for example, differentiate into osteoblasts when cultured on stiff matrices, while on intermediate matrices into myoblasts and on very soft matrices into neural-type cells. Cells growing in a tissue respond and differentiate depending on the microenvironment they experience. On the other hand, MSCs are known to display certain surface markers that are used to define their phenotype.
The goal of this project is to study and exploit the impact of cellular mechanics on cell differentiation and tissue growth. In a bottom-up approach we will use mechanically characterized and preselected mesenchymal stem cells (MSCs) and observe their behavior on synthetic 2D and 3D microenvironments with well-defined extracellular matrix (ECM). Here we want to investigate to what extend this mechanical pre-defined state of MSCs determine their lineage regardless of the mechanical properties of the ECM on which these cells are grown. In other words, whether cell mechanics is already a determinant for cell commitment.
We will employ a combination of contact and label-free techniques that allow for mechanical sampling and isolation of cells at a rate of several hundreds to thousands per second. We will employ microfluidic techniques and probe cells with low invasive hydrodynamic forces exerted by the external flow field. More precisely, we follow two complement strategies.
- Sorting in bulk by hydrodynamic (lift forces), deterministic lateral displacement (DLD) and acoustic filtering to isolate cells
- Determine single cellular mechanics using AFM and micropipette aspiration (MP)
We will investigate these mechanically selected cells on hydrogel environments to determine how MSCs with differing stiffness signatures interact and with soft, stiff and rigid ECM mimics.
Contact: Thomas.Franke@glasgow.ac.uk
Rapid Test for Precision Treatment of Microbial Infection - Prof. D. Cumming
Supervisors
David Cumming, Chunxiao Hu, and Mike Barrett (School of Infection and Immunity)
Description
Antimicrobial resistance (AMR) threatens to reverse the great strides in medicine that have accrued in the last 70 years. In addition to the concern about the re-emergence of bacterial infections as a major cause of death, with diseases such as typhoid, cholera and tuberculosis becoming commonplace, routine operations may become impossible due to the risk of infection and even childbirth will revert to the dangerous process it once was. Detecting AMR is critical to our ability to combat the problem. Currently two broad areas permit detection of drug resistant bacteria. On the one hand, phenotypic tests that follow the ability of bacteria to grow in the presence of antibiotics or not are widely used. Genetic tests that seek genes known to be associated with resistance have become more common as such genes are discovered and collated in sequence databases enabling detection. Arguably, phenotypic tests retain the advantage of reporting on resistance even where genetic mechanisms are not known. Recent advances in electronic engineering and microfluidics offer the potential to create portable devices that can detect antimicrobial resistant bacteria in a fraction of the time required using conventional methodologies. Here we propose to exploit microfluidic methods of sorting bacteria and electronic methods to detect viability of bacteria in the presence of antimicrobials to produce a new generation of antimicrobial resistance detecting devices that could underpin global efforts to combat this problem.
Contact: David.Cumming.2@glasgow.ac.uk