Parasitology PhD/iPhD/MSc (Research)
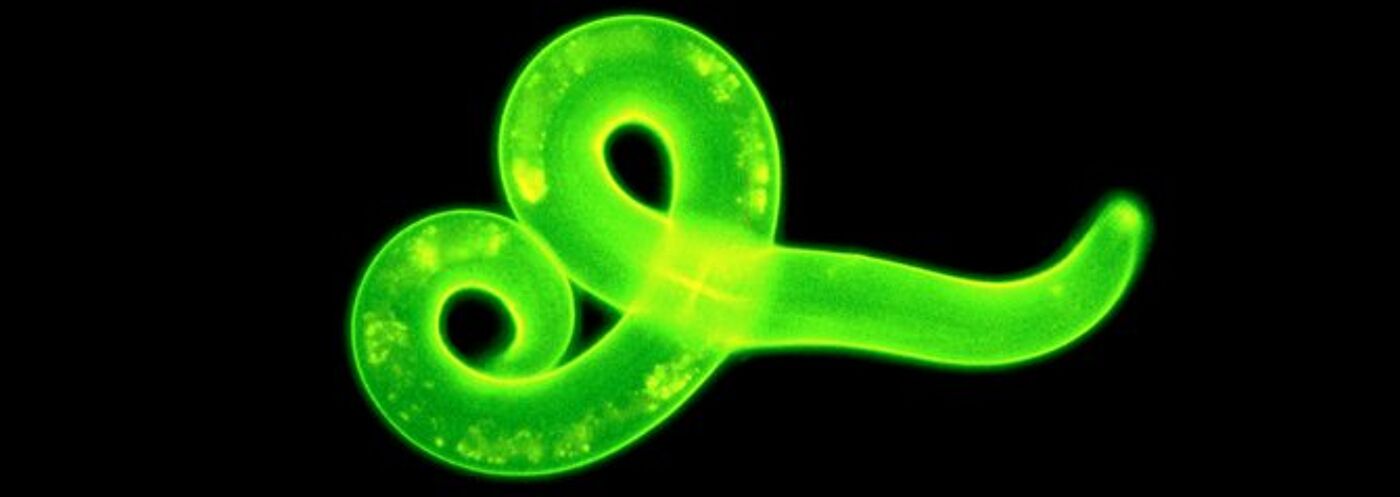
Our portfolio covers many aspects of parasitology research from molecules to cells and whole organisms to populations. Research activities are based around skills in molecular biology, biochemistry, immunology, epidemiology, population genetics and mathematical modelling and involve both laboratory-based projects and fieldwork.
- PhD: 3-4 years full-time; 5 years part-time;
- MSc (Research): 1 year full-time; 2 years part-time;
- IPhD: 5 years full-time;
Research projects
+++
Investigating the cell biology of Neoparamoeba perurans, a causative agent of Amoebic Gill Disease, and its endosymbiont kinetoplastid, Perkinsela.
Supervisors: Tansy Hammarton, Martin Llewellyn
Summary: Amoebic gill disease (AGD), caused by the opportunistic amoebozoan parasite Neoparamoeba perurans, is a major disease in salmonid aquaculture [1-3] and there is an urgent need to mitigate the impact parasitic infections have on both fish welfare and sustainable growth in the sector. Development of vaccine technology over the last 20 years has dramatically reduced losses associated with viral and bacterial disease. However, the industry remains beset by the biological threat posed by parasitic infections, including caligid sea lice as well as AGD, which are causing intolerable losses (c.20% annually). AGD is a primary driver of proliferative gill inflammation which impairs the respiratory capacity of fish leading to asphyxia, respiratory acidosis, acute hypertension, circulatory collapse and death [4]. Further, gill inflammation driven by exposure to micro-cnidarian blooms, for example, increases N. perurans gill colonisation and the prevalence of AGD at farm sites [5].
Treatment options for AGD are currently extremely limited. However, it may be possible to exploit the unique biology of N. perurans in future drug development. Strikingly, within each N. perurans cell is an endosymbiotic kinetoplastid, named Perkinsela, on which it relies for essential metabolic processes [6, 7]. This is a secondary endosymbiosis that is unique among eukaryotes because it does not involve an originally photosynthetic symbiont. Genomic data indicate that the basic physiology of Perkinsela shares many biochemical features with other kinetoplastid pathogens of man and livestock, although due to its small genome size (9.5 Mb, <5,500 genes), it has reduced metabolic capacity [6]. Furthermore, while molecular data indicates that Perkinsela retains some key features of kinetoplastid biology, including polycistronic transcription, trans-splicing and RNA editing, it has lost the genes required for flagellum biogenesis and is therefore aflagellate [6, 8].
Despite its importance, the life cycle of N. perurans is not well understood, and little is known of how the amoeba or its endosymbiont Perkinsela replicate and divide throughout the life cycle. This project seeks to define the life and cell cycles of N. perurans and Perkinsela in detail using light, fluorescence and electron microscopy. In particular, whether the replication of N. perurans and Perkinsela are linked and/or interdependent will be investigated. Further, their genomes will be bioinformatically mined for orthologues of cell cycle protein kinases and other regulators to catalogue the cell cycle machinery and permit functional characterisation. Cell cycle inhibitors may be employed to investigate cell cycle checkpoints, and molecular approaches (e.g. fluorescent tagging, RNAi, CRISPR/Cas9 etc) will be developed to allow functional characterisation of individual cell cycle proteins. Key cell cycle kinases of interest will also be expressed recombinantly to allow activity assays to be developed, which could facilitate future screening of small molecule inhibitor libraries for compounds that exert antiparasitic effects either directly on N. perurans or indirectly by targeting Perkinsela.
It is envisaged that these studies will not only lead to a greater understanding of the intriguing cell biology of the N. perurans-Perkinsela symbiosis, but will identify potential novel drug targets and provide context to investigations of the mode of action of other experimental drugs being developed to treat AGD.
References
- Clark A, Nowak BF (1999) Field investigations of amoebic gill disease in Atlantic salmon, Salmo salar L., in Tasmania. Journal of Fish Diseases 22: 433-443. https://doi.org/10.1046/j.1365-2761.1999.00175.x
- Rodger H (2013) Amoebic gill disease (AGD) in farmed salmon (Salmo salar) in Europe. Fish Veterinary Journal 14: 16-26.
- Bustos PA,, et al. (2011) Amoebic gill disease (AGD) in Atlantic salmon (Salmo salar) farmed in Chile. Aquaculture 310: 281-288. https://doi.org/10.1016/j.aquaculture.2010.11.001
- Powell MD, et al (2015) Freshwater treatment of amoebic gill disease and sea-lice in seawater salmon production. Aquaculture 448: 18-28.https://doi.org/10.1016/j.aquaculture.2015.05.027
- Kintner A, Brierley AS (2018) Cryptic hydrozoan blooms pose risks to gill health in farmed North Atlantic salmon (Salmo salar). Journal Marine Biological Association of the UK 99: 539-550. https://doi.org/10.1017/S002531541800022X
- Tanifuji, G., Cenci, U., Moog, D. et al. (2017) Genome sequencing reveals metabolic and cellular interdependence in an amoeba-kinetoplastid symbiosis. Scientific Reports 7: 11688. https://doi.org/10.1038/s41598-017-11866-x
- Nowak BF, Archibald JM. (2018) Opportunistic but lethal: the mystery of Paramoebae. Trends in Parasitology 34: 404-419. doi: https://doi.org/10.1016/j.pt.2018.01.004
- David, V., Flegontov, P., Gerasimov, E., Tanifuji, G., Hashimi, H., Logacheva, M. D., Maruyama, S., Onodera, N. T., Gray, M. W., Archibald, J. M., & Lukeš, J. (2015). Gene loss and error-prone RNA editing in the mitochondrion of Perkinsela, an endosymbiotic kinetoplastid. mBio 6: e01498-15. https://doi.org/10.1128/mBio.01498-15
Further information
---
+++
Understanding coordination of DNA replication initiation in the African trypanosome
Supervisor: Richard McCulloch
Summary: DNA replication is a central cellular reaction, necessary for genome transmission and the propagation of life. To date, structural and mechanistic studies on DNA replication have focused mainly on a small number of eukaryotes, revealing conserved machinery and reactions. However, emerging data from Trypanosoma brucei, a diverged eukaryotic microbe and major parasite, suggest the machinery, timing and regulation of DNA replication initiation may be distinct from this generalized model.
We hypothesise that assembly of the T. brucei DNA replication initiation machinery is deferred from the G1 phase of the cell cycle, as seen in all eukaryotes so far studied, and instead occurs during S phase. We will test this hypothesis through four objectives:
- DNA replication initiates in eukaryotes through the binding of a six subunit Origin Recognition Complex (ORC) to genomic loci termed origins. Previous data suggest that T. brucei ORC deviates from the conventional six-subunit architecture. We will test if this deviation is due to the action of a newly discovered, novel T. brucei ORC subunit that is only found in close relatives of T. brucei.
- Once bound to origins, ORC recruits the Minichromosome Maintenance (MCM) replicative helicase in G1, a reaction guided by two mediator factors, Cdc6 and Cdt1. To date, no discrete Cdc6-like protein has been described in T. brucei and so we will test if this activity resides in a protein termed TbORC1B, whose expression is limited to S phase.
- In other eukaryotes, MCM is assembled and recruited to ORC on origins in G1 of the cell cycle. We will test recent data suggesting that at least some subunits of MCM are only expressed in S phase, and if this means that MCM-ORC interaction is deferred until this cell cycle stage.
- No work has yet described a Cdt1-like mediator in T. brucei. We will test if this activity resides in a recently discovered, highly diverged Cdt1-related factor, including whether this activity is limited to S phase.
---
+++
Using microfluidics and flow cytometry to develop novel diagnostics for Leishmaniasis
Supervisors: Tansy Hammarton, Melanie Jimenez
Summary: The Leishmaniases are a group of neglected tropical diseases caused by >20 species of Leishmania parasites that are spread by >90 species of phlebotomine sand flies. 1 billion people in Africa, Asia, the Americas and the Mediterranean are at risk, with the poor and malnourished being disproportionately affected. >1 million new symptomatic cases are thought to occur annually (https://www.who.int/news-room/fact-sheets/detail/leishmaniasis) with symptoms ranging from disfiguring skin lesions (cutaneous leishmaniasis, CL), destruction of mucous membranes (mucocutaneous leishmaniasis, MCL) and visceral disease (VL) which is fatal in ~95% cases without treatment. However, it may take weeks to months after being bitten by an infected sand fly for symptoms to manifest, and many infected patients do not seem to develop symptoms [1]. In the absence of a vaccine, early diagnosis and prompt effective treatment are important to reduce the morbidity and mortality associated with Leishmania infection as well as to reduce the transmission and prevalence of leishmaniasis. With environmental and climate changes causing displacement of people from Leishmania-endemic areas and likely to result in migration of sand fly vectors northwards into new, naive areas, there is an urgent need to increase surveillance and enable early diagnosis of Leishmania infections to identify and reduce reservoirs that could sustain infection and transmission in these areas [2,3].
Leishmania promastigote forms are introduced into the skin of mammalian hosts when an infected sand fly bites. There they are taken up by host neutrophils and dermal tissue-resident macrophages, as well as some monocytes and monocyte-derived dendritic cells, where they differentiate to amastigotes and replicate. Apoptotic infected neutrophils may also be subsequently taken up by macrophages, increasing the infected macrophage population [4]. There is a plethora of methods used to diagnose leishmaniasis, ranging from observing clinical symptoms (which can be variable, non-specific and/or atypical if a patient is co-infected with e.g. HIV, malaria or pulmonary tuberculosis) to parasitological methods (the gold standard) involving direct visualisation of the parasite in splenic, bone-marrow or lymph node aspirates or following culturing from the aspirates or blood, as well as immunological and molecular methods [5]. There are disadvantages to all of these methods. Some have low sensitivity and/or specificity or only work for some species of Leishmania or may not be able to distinguish between past and present infection. For others, there are issues of the tests requiring specialised equipment and/or expertise meaning they are costly and time-consuming to perform. Finally, some rely on obtaining aspirates from e.g. bone marrow or spleen, liver biopsies or lesion scrapings, which can be painful or even dangerous for the patient.
Being able to diagnose Leishmania infection rapidly and accurately from a simple blood sample would be highly beneficial and might also allow infected but asymptomatic patients to be identified so that they can be closely monitored and treated promptly if they subsequently develop symptoms. It would also allow large scale screening for infections and cases, aiding epidemiological and surveillance studies. Studies have shown that Leishmania parasites can be cultured or detected microscopically from blood samples, buffy coat preparations and isolated peripheral blood mononuclear cells in patients with CL and VL, but that these methods of detection lack sensitivity [6,7]. Microfluidic sorting of blood or purified blood fractions using small spiral channels may offer a route to enriching for Leishmania-infected cells to increase the sensitivity of diagnosis. Microfluidic inertial-focussing is a simple, rapid, portable and high throughput (>106 cells/min) method that enables separation of cells based on differences in their shape, size and deformability [8,9]. Currently, we are optimising this method to separate different cell cycle stages of Leishmania promastigote parasites. This proposed PhD project will complement this work by investigating whether microfluidic sorting can be adapted to sort Leishmania-infected white blood cells from non-infected cells. Infection of host cells by parasites such as Plasmodium spp. and Trypanosoma cruzi is known to increase host cell stiffness, in part due to molecular changes that the parasite induces, and in part due to the physical presence of the parasite itself [10,11]. While changes to host cell deformability following infection with Leishmania parasites have not been investigated in detail, studies have shown that Leishmania spp. alter the composition of the macrophage plasma membrane, suggesting that changes in stiffness are likely [12,13]. As such, by optimising the dimensions of microfluidic spiral channels, we believe it should be possible to exploit alterations in stiffness to separate Leishmania-infected white blood cells from uninfected cells.
A combination of deformability and imaging flow cytometry and microscopy will be employed to investigate changes in the deformability, size and shape of white blood cells such as neutrophils, monocytes and macrophages upon infection by a range of Leishmania species (e.g. L. mexicana, L. major, L. infantum etc.). This information will shed light on the Leishmania infection process, allowing hypotheses about the molecular changes to the host cell induced by the parasite to be developed and tested using e.g. proteomics or lipidomics technologies, and will enable the optimisation of the dimensions and outlets of microfluidic spiral devices to separate infected white blood cells from non-infected cells. It is hoped this will provide proof of principle for a novel diagnostic method for leishmaniasis.
References
- Singh O, Hasker E, Sacks D, et al. (2014). Asymptomatic Leishmania infection: a new challenge for Leishmania control, Clin Infect Dis 58: 1424–1429. https://doi.org/10.1093/cid/ciu102
- Chalghaf B, Chemkhi J, Mayala B et al. (2018). Ecological niche modeling predicting the potential distribution of Leishmania vectors in the Mediterranean basin: impact of climate change. Parasit Vectors 11, 461. https://doi.org/10.1186/s13071-018-3019-x
- Curtin JM, & Aronson NE (2021). Leishmaniasis in the United States: emerging issues in a region of low endemicity. Microorganisms, 9: 578. https://doi.org/10.3390/microorganisms9030578
- Chaves MM, Lee SH, Kamenyeva O et al. (2020). The role of dermis resident macrophages and their interaction with neutrophils in the early establishment of Leishmania major infection transmitted by sand fly bite. PLoS Pathog 16: e1008674. https://doi.org/10.1371/journal.ppat.1008674
- Thakur S, Joshi J & Kaur, S (2020). Leishmaniasis diagnosis: an update on the use of parasitological, immunological and molecular methods. J Parasit Dis 44: 253–272. https://doi.org/10.1007/s12639-020-01212-w
- Nakkash-Chmaisse H, Makki R, Nahhas G et al. (2011). Detection of Leishmania parasites in the blood of patients with isolated cutaneous leishmaniasis. Int J Infect Dis 15: e491-4. https://doi.org/10.1016/j.ijid.2011.03.022
- Diro E, Yansouni CP, Takele Y et al. (2017). Diagnosis of visceral Leishmaniasis using peripheral blood microscopy in Ethiopia: a prospective Phase-III study of the diagnostic performance of different concentration techniques compared to tissue aspiration. Amer J Trop Med Hyg 96: 190–196. https://doi.org/10.4269/ajtmh.16-0362
- Jimenez M, Miller B, Bridle HL (2017). Efficient separation of small microparticles at high flowrates using spiral channels: application to waterborne pathogens. Chem Eng Sci 157: 247–54.
- Guzniczak E, Otto O, Whyte G et al. (2020) Deformability-induced lift force in spiral microchannels for cell separation. Lab Chip 20: 614-625.
- Hosseini SM & Feng JJ (2012). How malaria parasites reduce the deformability of infected red blood cells. Biophys J 103: 1–10. https://doi.org/10.1016/j.bpj.2012.05.026
- Mott A, Lenormand G, Costales J et al. (2009). Modulation of host cell mechanics by Trypanosoma cruzi. J Cell Physiol 218: 315-22. https://doi.org/10.1002/jcp.21606
- Forero M, Marín M, Corrales A et al. (1999). Leishmania amazonensis infection induces changes in the electrophysiological properties of macrophage-like cells. J. Membrane Biol. 170: 173–180. https://doi.org/10.1007/s002329900547
- Ghosh M, Roy K, Das Mukherjee D et al. (2014). Leishmania donovani infection enhances lateral mobility of macrophage membrane protein which is reversed by liposomal cholesterol. PLoS Negl Trop Dis 8: e3367. https://doi.org/10.1371/journal.pntd.0003367
---
Overview
Parasites are fascinating organisms, because of their capacity to live and replicate within the host environment. How parasites adapt and survive is the focus of much study with the potential to generate new and important discoveries that can impact upon control. Most organisms harbor parasites and much of our research is aimed at understanding how parasites cause disease and how best to control disease in both humans and animals. Improving upon existing treatments and understanding mechanisms of drug resistance are important aspects of this work. In addition, the epidemiology, ecology and population genetics of parasitic pathogens are important areas of research that significantly impact upon transmission and control.
Our research portfolio covers a range of tropical parasites that cause important diseases, including Plasmodium ssp (malaria), Trypanosoma ssp (sleeping sickness), Leishmania ssp (leishmaniasis) and Theileria (East coast fever/theileriosis), along with filarial worms, the cause of elephantiasis. We also study parasites that are endemic in the UK such as Toxoplasma gondii and important gastro-intestinal parasites of livestock that cause significant economic loss to the agricultural industry and are important for global food security. Many important parasites are transmitted by vectors, and we have growing strengths in vector biology, most notably mosquitoes and ticks. We aim to apply our findings to informing control programmes and to translate our findings into better diagnostics, drugs and vaccines.
Students undertake individual research projects in the area of expertise of their supervisor(s), although many projects on offer are interdisciplinary.
Your choice of projects is diverse, reflecting the range of expertise of potential supervisors. The University of Glasgow provides an excellent environment for parasitology research, housing the largest group of parasitologists in the UK, studying all aspects of parasitic disease from gene to population. Parasitology is housed within the School of Infection and Immunity and the School of Biodiversity, One Health, and Veterinary Medicine. In addition, many of the group are members of the Wellcome Centre for Integrative Parasitology. The resources available provide the opportunity for excellent and cutting edge training in many different areas. These include molecular biology, biochemistry, ecology, epidemiology, mathematical modelling, bioinformatics, genetics, cell biology (including advanced in vitro and in vivo imaging), immunology and polyomics (genomics, transcriptomics, proteomics, metabolomics etc). Many projects are laboratory-based in up-to-date facilities with excellent research resources. Interdisciplinary research is a key aspect of our approach and we have many collaborators both within the university and externally. Some projects involve considerable amounts of fieldwork in the UK or overseas.
Specific areas of interest include:
- molecular basis of sexual development in Plasmodium (Waters)
- genetics and biology of the interactions between P. falciparum and the mosquito vector (Ranford-Cartwright)
- ecology and behaviour of malaria vectors (Ferguson)
- cell division in trypanosomes (Hammarton)
- homologous recombination, DNA repair pathways and antigenic variation in T. brucei (McCulloch)
- african trypanosomes and their interactions with their hosts (MacLeod)
- neuropathology of African trypanosomiasis (Rodgers)
- biogenesis of the mitochondrion and apicoplast in T. gondii (Sheiner)
- immune regulation in vivo in relation to parasitic infection (Brewer, Garside)
- fitness costs of the immune response and wild immunology (Babayan)
- imaging the immune response to parasites in vivo (Brewer)
- mathematical modelling of host-parasite systems (Matthews)
- epidemiology of zoonotic pathogens in Africa (MacLeod)
- mechanisms of drug resistance (protozoan, nematode, ectoparasites) (Barrett, de Koning, Jonsson)
- the role of membrane transporters in parasite virulence and drug susceptibility (de Koning)
- development of new lead compounds and vaccines for parasitic diseases (protozoan and nematode) (Barrett, Page, Britton)
- use of C. elegans as a model for understanding gene function in parasitic nematodes (Britton, Page)
- microRNAs and their functions in parasitic nematodes (Britton)
Study options
PhD
- Duration: 3/4 years full-time; 5 years part-time
Individual research projects are tailored around the expertise of principal investigators.
Integrated PhD programmes (5 years)
Our Integrated PhD allows you to combine masters level teaching with your chosen research direction in a 1+3+1 format.
International students with MSc and PhD scholarships/funding do not have to apply for 2 visas or exit and re-enter the country between programmes. International and UK/EU students may apply.
Year 1
Taught masters level modules are taken alongside students on our masters programmes. Our research-led teaching supports you to fine tune your research ideas and discuss these with potential PhD supervisors. You will gain a valuable introduction to academic topics, research methods, laboratory skills and the critical evaluation of research data. Your grades must meet our requirements in order to gain entry on to your pre-selected PhD research project. If not, you will have the options to pay outstanding MSc fees and complete with masters degree only.
Years 2, 3 and 4
PhD programme with research/lab work, completing an examinable piece of independent research in year 4.
Year 5
Thesis write up.
MSc (Research)
- Duration: 1 year full-time; 2 years part-time
Entry requirements
A 2.1 Honours degree or equivalent.
English language requirements
For applicants from non-English speaking countries, as defined by the UK Government, the University sets a minimum English Language proficiency level.
International English Language Testing System (IELTS) Academic and Academic Online (not General Training)
- 6.5 with no subtests under 6.0
- IELTS One Skill Retake Accepted
- Tests must have been taken within 2 years 5 months of start date. Applicants must meet the overall and subtest requirements using a single test.
Common equivalent English language qualifications for entry to this programme
TOEFL (ibt, mybest or athome)
- 90 overall with Reading 20; Listening 19; Speaking 19; Writing 21
- Tests must have been taken within 2 years 5 months of start date. Applicants must meet the overall and subtest requirements, this includes TOEFL mybest.
Pearsons PTE Academic
- 59 with minimum 59 in all subtests
- Tests must have been taken within 2 years 5 months of start date. Applicants must meet the overall and subtest requirements using a single test.
Cambridge Proficiency in English (CPE) and Cambridge Advanced English (CAE)
- 176 overall, no subtest less than 169
- Tests must have been taken within 2 years 5 months of start date. Applicants must meet the overall and subtest requirements using a single test.
Oxford English Test
- 7 overall with no subtest less than 6
- Tests must have been taken within 2 years 5 months of start date. Applicants must meet the overall and subtest requirements using a single test.
LanguageCert Academic SELT
- 70 overall with no subtest less than 60
- Tests must have been taken within 2 years 5 months of start date. Applicants must meet the overall and subtest requirements using a single test.
Password Skills Plus
- 6.5 overall with no subtest less than 6.0
- Tests must have been taken within 2 years 5 months of start date. Applicants must meet the overall and subtest requirements using a single test.
Trinity College Tests
- Integrated Skills in English II & III & IV: ISEII Distinction with Distinction in all sub-tests
- Tests must have been taken within 2 years 5 months of start date. Applicants must meet the overall and subtest requirements using a single test.
University of Glasgow Pre-sessional courses
- Tests are accepted for 2 years following date of successful completion.
Alternatives to English Language qualification
- Degree from majority-English speaking country (as defined by the UKVI including Canada if taught in English)
- students must have studied for a minimum of 2 years at Undergraduate level, or 9 months at Master's level, and must have completed their degree in that majority-English speaking country within the last 6 years.
- Undergraduate 2+2 degrees from majority-English speaking country (as defined by the UKVI including Canada if taught in English)
- students must have completed their final two years study in that majority-English speaking country within the last 6 years.
For international students, the Home Office has confirmed that the University can choose to use these tests to make its own assessment of English language ability for visa applications to degree level programmes. The University is also able to accept UKVI approved Secure English Language Tests (SELT) but we do not require a specific UKVI SELT for degree level programmes. We therefore still accept any of the English tests listed for admission to this programme.
Pre-sessional courses
The University of Glasgow accepts evidence of the required language level from the English for Academic Study Unit Pre-sessional courses. We would strongly encourage you to consider the pre-sessional courses at the University of Glasgow's English for Academic Study (EAS) Unit. Our Pre-sessional courses are the best way to bring your English up to entry level for University study. Our courses give you:
- direct entry to your University programme for successful students (no need to take IELTS)
- essential academic skills to help you study effectively at University
- flexible entry dates so you can join the right course for your level.
For more detail on our pre-sessional courses please see:
We can also consider the pre-sessional courses accredited by the below BALEAP approved institutions to meet the language requirements for admission to our postgraduate taught degrees:
- Heriot Watt
- Kingston Upon Thames
- Middlesex University
- Manchester University
- Reading University
- Edinburgh University
- ST Andrews University
- UCL
- Durham.
Fees and funding
Fees
2025/26
- UK: £5,005
- International & EU: £31,800
Prices are based on the annual fee for full-time study. Fees for part-time study are half the full-time fee.
Irish nationals who are living in the Common Travel Area of the UK, EU nationals with settled or pre-settled status, and Internationals with Indefinite Leave to remain status can also qualify for home fee status.
Alumni discount
We offer a 20% discount to our alumni on all Postgraduate Research and full Postgraduate Taught Masters programmes. This includes University of Glasgow graduates and those who have completed Junior Year Abroad, Exchange programme or International Summer School with us. The discount is applied at registration for students who are not in receipt of another discount or scholarship funded by the University. No additional application is required.
Possible additional fees
- Re-submission by a research student £540
- Submission for a higher degree by published work £1,355
- Submission of thesis after deadline lapsed £350
- Submission by staff in receipt of staff scholarship £790
Depending on the nature of the research project, some students will be expected to pay a bench fee (also known as research support costs) to cover additional costs. The exact amount will be provided in the offer letter.
Funding
The IPhD is not supported by University of Glasgow Scholarship/Funding
- BBSRC Doctoral Training Partnerships
- External funding information
Support
The College of Medical, Veterinary and Life Sciences Graduate School provides a vibrant, supportive and stimulating environment for all our postgraduate students. We aim to provide excellent support for our postgraduates through dedicated postgraduate convenors, highly trained supervisors and pastoral support for each student. Through their research interests in drug development, vaccines and diagnostics, many of our project supervisors have strong links with industry.
Our overarching aim is to provide a research training environment that includes:
- provision of excellent facilities and cutting edge techniques
- training in essential research and generic skills
- excellence in supervision and mentoring
- interactive discussion groups and seminars
- an atmosphere that fosters critical cultural policy and research analysis
- synergy between research groups and areas
- extensive multidisciplinary and collaborative research
- extensive external collaborations both within and beyond the UK
- a robust generic skills programme including opportunities in social and commercial training
Research environment
If you study with us, you will join a large community of postgraduate taught and research students. Our Schools bring together world-leading basic, applied, clinical and translational researchers to study infection with a focus on the viral, parasitic and bacterial pathogens of both humans and animals, and immunology and inflammation with a focus on chronic inflammatory diseases.
Despite the continual development of new therapies, antibiotics and vaccines, chronic inflammatory and infectious diseases still pose persistent health threats. We aim to:
- understand the basic science of the immune systems and how the immune system can inturn affect disease outcome understand the biology of parasites, viruse and bacteria and the interactions with their hosts, that in turn leads to high levels of infectious diseases worldwide
- develop therapies (drugs and vaccines) targeted on these processes
- explore new treatments and strategies in clinical and translational medicine
Research centres
- MRC-University of Glasgow Centre for Virus Research
- Wellcome Centre for Integrative Parasitology
- ARUK Centre for Experimental Arthritis Research
Resources
Our excellent facilities underpin a bench to bedside approach that will equip you with training complementary to a range of career options, and you can tailor your study pathway to the precise aspects of infection and immunology that suit your objectives. Facilities include:
- core facilities in fluorescence activated cell sorting analysis
- histology and state-of-the-art imaging.
- IVIS imaging system
- high content screening microscopy
- mass spectrometry
- an X-ray capable FX Pro bioluminescence imaging system
- a protein purification service
- a wide range of molecular, immunological and biochemical analysis tools
How to apply
Identify potential supervisors
All postgraduate research students are allocated a supervisor who will act as the main source of academic support and research mentoring. You must identify a potential supervisor and contact them to discuss your research proposal before you apply. Please note, even if you have spoken to an academic staff member about your proposal you still need to submit an online application form.
IPhD & research projects
IPhD Option A
Applicants do not need to contact a supervisor. You will choose from a list of IPhD projects and each project has named supervisors linked to that project.
IPhD Option B
You will submit a research proposal of approximately 1000 words. The proposal must include:
- a straightforward, descriptive, and informative title
- the question that your research will address
- an account of why this question is important and worth investigating
- an assessment of how your own research will engage with recent research in the field
- a brief account of the methodology and approach you will take.
Based on your proposal, your supervisor will choose an MSc programme that aligns with your research interests.
You will need to contact a supervisor prior to application, using our search to identify a suitable supervisor.
Research projects
If you are seeking to apply for any research project, you can identify this within your application to the PhD programme. Please ensure that you highlight the title of the research project you are interested in on your application.
Gather your documents
Before applying please make sure you gather the following supporting documentation:
- Final or current degree transcripts including grades (and an official translation, if needed) – scanned copy in colour of the original document.
- Degree certificates (and an official translation, if needed): scanned copy in colour of the original document.
- Two references on headed paper and signed by the referee. One must be academic, the other can be academic or professional (except IPhD applicants, where only one academic or professional reference is required). References may be uploaded as part of the application form or you may enter your referees' contact details on the application form. We will then email your referee and notify you when we receive the reference.
- Research proposal (if applying for PhD or MScR), CV, samples of written work as per requirements for each subject area.
Contact us
If you require assistance before you apply: mvls-gradschool@glasgow.ac.uk
After you have submitted your application: Admissions Enquiries form
More information
Our research environment
Induction
- Getting started with PGR development: how postgraduate researchers are welcomed into our community