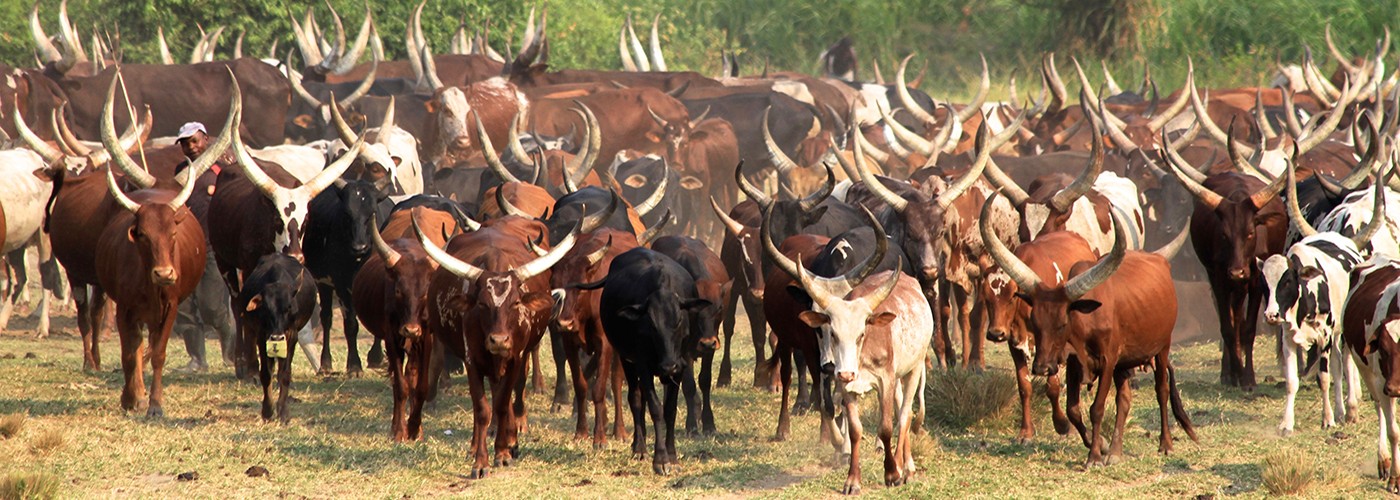
Animal African Trypanosomiasis
Scientists at Glasgow as well as Strathclyde, Edinburgh and Liverpool School of Tropical Medicine, are collaborating on projects to improve control of a long-neglected parasitic disease.
Animal African Trypanosomiasis (AAT), also known as Nagana, is a devastating parasitic disease that affects domestic animals including cattle, sheep, goats and horses in 40 countries in sub-Saharan Africa.
The disease is a major constraint to livestock production with three billion dollars of lost animal productivity attributed to it. These economic losses are endured by those in some of the poorest parts of the world, namely small-scale subsistence farmers and rural communities in Africa who suffer diminished meat and milk yields as well as a reduction in draught animal production.
AAT is caused by microscopic blood-dwelling parasites called trypanosomes which are transmitted by the bite of a tsetse fly, which acts as the biological vector. Once their blood is infected, animals suffer from fever, weakness, lethargy and anaemia which leads to weight loss as well as fertility and milk reduction. Eventually, the animal may die. Africa has historically endured the greatest burden, but parts of South America and South-East Asia are also affected where the disease is transmitted by other biting flies.
The disease has a human variant, known as human African trypanosomiasis (HAT) or sleeping sickness, which is a debilitating and often fatal disease that affects thousands of people living in rural areas every year.
Trypanosomiasis has afflicted Africa for centuries. The celebrated nineteenth century explorer and medical missionary David Livingstone noted during his extensive travels across Africa that the tsetse fly and trypanosomiasis (or fly disease as he called it back then) were major hurdles to the development of the continent.
The disease remains a huge problem and hindrance to development today. For a long time, research into AAT was neglected due to a lack of resources and political motivation, but in recent years there has been a renewed focus on tackling the disease, with Glasgow playing a key role.
Controlling AAT
Three main species of trypanosomes are the cause of AAT: Trypanosoma congolense, Trypanosoma vivax and, to a lesser degree, Trypanosoma brucei. Vaccinating against AAT is futile due to the sophisticated and evasive nature of the parasite. The parasites are shrouded in a thick glycoprotein coat, which they can intermittently change, resulting in the immune system being in a constant state of catch-up to identify the ever-changing parasites.
Therefore many livestock keepers in affected areas regularly use insecticides to protect their livestock from tsetse flies and trypanocidal drugs to control the disease.
For many years, there have been several trypanocidal drug compounds available which work both prophylactically to prevent AAT (isometamedium) and curatively to rid an animal of the disease (diminazene). However, these drugs are not always effective. This may be due to losing efficacy as a result of increasing drug resistance in the parasites. In addition, there is a market in ineffective counterfeit versions of these drugs which farmers are buying because they are cheaper. New injectable drug compounds are therefore desperately needed to curb the disease, as well as a better understanding of other reasons why the drugs are not always effective.
University of Glasgow researchers are involved with several projects to address these issues.
Understanding treatment failure and resistance
Scientists from University of Glasgow, University of Edinburgh, Liverpool School of Tropical Medicine, Vector and Vector-borne Disease Institute in Tanzania (VVBDI), and the National Institute of Medical Research, Tanzania (NIMR) are working together on a project funded by BBSRC and Galvmed to understand the role that trypanocide resistance is playing in treatment failures for AAT. The project combines several components:
Harriet Auty and PDRA Shauna Richards, veterinary epidemiologists based at University of Glasgow, are working with VVBDI and NIMR to identify and quantify the reasons for treatment failure in Tanzania, and assess the impact of resistant trypanosomes.
Mike Barrett’s group (University of Glasgow) along with Liam Morrison and PDRA Pete Steketee (Roslin Institute, University of Edinburgh), are working to identify mechanisms and markers of resistance of T. congolense to isometamidium, one of the drugs commonly used for AAT.
Louise Matthews and PDRA Shaun Keegan (University of Glasgow) are developing mathematical models of AAT transmission, based on data generated in the field and laboratory parts of the project, to understand how resistance can emerge and spread, as well as its impact on AAT control in the future.
Together these findings aim to develop more sustainable control options for AAT, as well as informing future new drug development.
Developing new drugs
GALVmed, a not-for-profit veterinary health charity based in Scotland that develops vaccines and drugs for livestock diseases, have undertaken work on AAT since 2011.
In 2016, GALVmed began working with researchers at the University of Strathclyde, the Roslin Institute at the University of Edinburgh and the University of Glasgow on the development of new drugs that are effective against the trypanosomes T.congolense and T.vivax, as these two parasites are generally found in the same geographical areas. The research also aims to better understand the biology of these two key trypanosomes and their responses to drugs.
In addition to support from GALVmed, the researchers received £592,314 of funding from the BBSRC.
Professor Mike Barrett, Professor of Biochemical Parasitology at the Wellcome Centre for Integrative Parasitology, is leading efforts within the University of Glasgow branch of the project along with Professor Harry De Koning.
Evaluating new drug compounds
One of the main aims of the project is to test a new series of injectable drug compounds which have been invented and developed by a group led by Professor Colin Suckling in the Chemistry department at the University of Strathclyde. This class of compounds, called “minor groove binders”, bind themselves to the parasite’s DNA, specifically the minor grooves within the double helical structure of the DNA.
The compounds mimic a natural product called distamycin, that was observed to have anti-microbial properties many years ago. Its peptide-like molecular structure was easy to replicate in the lab. Once replicated, these structures could be strung together and configured in different ways to find the most potent compounds against various microbes.
Professor Barrett explains: “It's a bit like molecular lego. What you can do is build these chains and see which are the most active against any microbial agent you’re interested in. With a whole bunch of these things you can test which ones are most potent, and if any common features stand out in those that are most potent. You then keep on iteratively juggling the structure and getting more and more potency.”
In addition to trypanosomes, this chemical library has produced compounds that are active against Malaria, bacterial infections such as Clostridium difficile, as well as various viruses.
“It’s a remarkable series of compounds and because it’s so modular, it means Colin can keep on shuffling them to find which group of molecules work best against trypanosomes and which are best against certain bacteria. He’s in some ways got a gold mine of chemical diversity from which he can keep taking out compounds.”
Left: a representation of a model of a Strathclyde-MGB (green, white, blue, red, yellow) bound to DNA (blue) in the minor groove. Right: Trypanosoma congolense being treated with a fluorescent S-MGB, which localises in the parasite's DNA.
Having created these compounds, the next step was for the Glasgow group to test their efficacy against trypanosomes and try to unlock the mystery as to exactly how they work and why they appear to kill trypanosome cells better than mammalian cells.
“We’ve got DNA with a minor groove and these compounds bind to a minor groove. So we’re trying to understand exactly how they kill trypanosomes selectively over mammalian cells.” says Professor Barrett. “We need to find out which features of the drug make it better against parasites and less good against mammalian cells to get that selectivity. So we collect the data on how they kill mammalian cells and how they kill trypanosomes, then feed that data back to Colin at Strathclyde to help him to make adjustments in order to achieve more anti-parasite activity and less anti-mammal activity.”
This evaluation of the compounds has been relatively successful so far with some potent compounds and some selective compounds having been identified, but there is a still a little way to go until the researchers identify the perfect selectivity to kill the parasites: “We’re not quite at the position yet where we’ve got the perfect marriage between super activity against the parasites and super inactivity against the mammal, but we do think we’re getting there.”
The researchers are also trying to better understand how these new drugs work by studying the internal functioning of the parasite. Using metabolomics technology at Glasgow Polyomics, a University of Glasgow facility which uses state of the art technology to sequence genomes and also quantify all of a cell’s chemical composition, Professor Barrett and his team are investigating whether, as well as binding to parasite DNA, the drugs are affecting parasite metabolism as well:
“So far it does look as if the drugs are mainly working by hitting DNA, but there must be some other strands to their specificity and we’re still not sure as to what they are. It may be to do with them more readily being taken up into parasites than they are into mammalian cells, but we haven’t got that proof yet.”
Professor Barrett has also sought to find out how readily the parasites might become resistant to these new drugs.
With the existing drugs available for AAT, the parasites have mutated in order to become resistant. By selecting the mutants, scientists can study the mutation process and understand the specifics of how the drug works on the parasite.
Investigating these new compounds has revealed resistance in them to be virtually non-existent. This is good news as it means that parasites are highly unlikely to develop resistance to these drugs in the field. However, the flip side to this is that the lack of resistance means the researchers have fewer leads into how the drugs work on the parasite, as it is the process of selecting for resistance that enables the researchers to understand exactly how the parasite’s resistance mechanism works. Professor Barrett explains further:
“When you select resistant parasites, you can simply sequence the genome of the parasite before you select for resistance and then sequence it again afterwards, and you often find a difference. You might find that they’ve changed a gene that encodes for the enzyme that is the target of the drug, or it might be the gene that encodes for a membrane transporter that brings the drug into the cell, or a protein on the cell membrane that binds to the drug and gets endocytosed. We were hoping that was going to work and from that perspective it’s bad news that it’s been difficult to get resistant lines. We haven’t enabled the parasite to tell us what’s going on.”
Though not essential to understand exactly how drugs work, it is helpful in that if the researchers understand how the drug works, they can fast track their next iterations enabling a quicker route to finding even better compounds in future.
Finding improved culture media
A second strand of the project is to create a better in vitro culture solution for the study of T.congolense and T.vivax. This is a solution that would contain all the nutrients, including sugars, lipids, amino acids and so on that the parasite needs to thrive outside its host. Culture media enable scientists to grow the parasite in a lab and subsequently easily and cheaply test new drug compounds.
For years, there has been an effective culture media for the study of T.brucei. For T.congolense, only some strains could be cultured whilst for T.vivax, it has proved incredibly difficult to grow the parasite in culture media at all.
The aim was to discover which mix of chemicals best mimic the conditions for the parasite to thrive inside of an animal.
“Liam Morrison and his group at the Roslin Institute at the University of Edinburgh are trying to find a really robust culture medium which will enable the parasites to be as close to their physiological state as they would be inside an animal” says Professor Barrett. “That’s where their biochemistry – which is what you end up hitting with a drug – is going to be most similar to what it’s going to be like in an animal.”
The Roslin team select chemicals to create new culture media which are then sent to Professor Barrett’s lab to test, in order to find out which elements of the solution are most effective in growing the parasite. A key part of Professor’s Barrett’s testing process is metabolomics, which enables him to simultaneously measure all chemicals in a complex mixture.
By measuring the chemicals in a culture media before a parasite is added to it, and then measuring the chemicals in it as the parasite begins to grow, it is possible to see which chemicals the parasites need in order to develop.
Previously, medium development was undertaken through trial and error – adding and subtracting various chemicals until eventually the parasite grows, but metabolomics enables the researchers to approach it more strategically.
“What metabolomics allows us to do is see is which chemicals from the medium the parasite is taking to turn its cells into polymers which then make up the parasite. This new technology enables us to make a rationally designed medium knowing what it is that the parasite wants and what it doesn’t want.”
Though a T.vivax culture is still proving difficult, as a result of this process, the team have now developed a medium which is able to support the growth of different T.congolense cell lines.
Breakthroughs
Two years into the project, the collaborating researchers have made steady progress with developing ever more potent compounds against the parasite, and in a predictable fashion. Two compounds in particular have proved to be highly potent against trypanosomes and tantalisingly close to having all features necessary to cure the disease.
Benefits for human disease from AAT control
Running in parallel to this lab-based research into new drug development, Glasgow researchers are carrying out field-based research in Tanzania looking at AAT control.
Edges of wilderness areas where wildlife reservoirs of HAT/AAT and tsetse populations coexist present a particular risk for disease transmission. Work by Dr Harriet Auty and partners around Serengeti, Tanzania suggests that the use of insecticide by livestock-keepers is preventing the spread of r-HAT in these areas. By treating livestock to prevent animal diseases, livestock-keepers are protecting local people from r-HAT.
While this discovery in Serengeti offers the exciting prospect of a One Health solution to r-HAT, it is not clear (i) how widespread pyrethroid use is in different livestock systems, (ii) how generally applicable this approach is, and (iii) what conditions make farmer-led control likely and effective.
A project called Enables: Enabling livestock keepers to control sleeping sickness, funded by BBSRC and DFID as part of the Zoonoses in Emerging Livestock Systems programme, is addressing these questions. The project combines mapping of tsetse populations with cattle sampling and collection of questionnaire data from livestock keepers on AAT control, to understand the effects of disease control of HAT risk and help formulate evidence-based policies and practices for the sustainable control of sleeping sickness.
Selected publications
- 'Assessing the effect of insecticide-treated cattle on tsetse abundance and trypanosome transmission at the wildlife-livestock interface in Serengeti, Tanzania', PLOS Neglected Tropical Diseases
- 'Geostatistical models using remotely‐sensed data predict savanna tsetse decline across the interface between protected and unprotected areas in Serengeti, Tanzania', Journal of Applied Ecology
Animal African Trypanosomiasis research in Tanzania
Researchers outline the issues with current AAT control in Tanzania, and what Glasgow scientists are doing to tackle them.